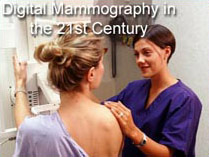
Digital Mammography in the 21st Century
Imaging research supported by the National Cancer Institute (NCI) is advancing on several fronts. Besides efforts to improve conventional and digital X-ray mammography, NCI also supports research for several other technologies such as magnetic resonance imaging (MRI), ultrasonography, positron emission tomography (PET), and single photon emission computed tomography (SPECT). Already, with these technologies, scientists can “see” biological processes taking place in living tissues such as blood flow, oxygen consumption, and glucose metabolism.
A major research effort is under way to capitalize on the abundant discoveries in cancer biology and create imaging technologies that can non-invasively detect and display the actual molecular events taking place in the body. Molecular imaging will allow researchers to detect altered gene products and tumor-specific receptors or enzymes. The ability to visualize molecular pathways involved in the development of tumors is expected to enable researchers to detect and stage tumors more easily, to select more effective treatments, and to monitor the effectiveness of new drugs. Information on the Biomedical Imaging Program at NCI is available at http://nci.nih.gov/bip/default.htm.
For fiscal year 2000, NCI spent $102 million on its imaging program. The exact figures for 2001 are not known, but at this time spending has reached $115 million. For the last few years, imaging has been identified as one of a handful of extraordinary scientific opportunities that hold the greatest potential to lead to major improvements in cancer management. Special funds targeted for imaging research were requested for the 2002 budget. (http://plan2002.cancer.gov/scpimaging.htm)
Active Areas of Breast Imaging Research
- Digital Mammography Display and Workstation Technology
Mammography creates images by passing x-rays through the breast. In conventional mammography the images are recorded on film whereas in digital mammography, the image is recorded as an electronic signal, stored in a computer, and displayed on a printed film or monitor. Current research is aimed at determining the best methods for obtaining and displaying digital images. Also under investigation are computer-aided detection and diagnosis programs to assist the physician in interpreting abnormal findings. See: http://researchportfolio.cancer.gov/, enter search term, ‘mammography.’
- Computed Tomography (CT)
As with conventional X-rays, CT images are created by passing X-rays though the body. However, unlike standard X-rays where the X-ray source is immobile, in CT, the X-ray source moves around the patient, and serial cross-sectional pictures of the patient are recorded. The data are then reconstructed into a 2- or 3-dimensional pictures by sophisticated computer programs. Because tissue contrast resolution is much greater in CT than in conventional X-rays, CT can detect very small difference in X-ray absorption. The result is that CT can clearly show the shape and exact location of organs, soft tissues, and bones. By injecting contrast material through a vein, the exquisite detail of CT scans allows the detection of very small and early abnormal changes in organs and soft tissues.
CT is the most commonly used technology in clinical trials. The American College of Radiology Imaging Network (ACRIN), the most recently created cooperative group (http://ctep.cancer.gov) funded and supported by NCI that manages clinical trials of imaging technologies as they relate to cancer, is conducting a broad spectrum of multi-institutional clinical trials on new and refined imaging tools. ACRIN has developed a large group of clinical research studies involving CT. (http://www.acrin.org)
- Magnetic Resonance Imaging
The images produced by MR are the result of radio signals emitted by specific atoms in a patient who is lying in a strong magnetic field. The process depends on the fact that the nuclei of certain elements (primarily hydrogen in the body’s water and fat) act like small, spinning bar magnets, and align with a strong magnetic field when placed in it. The radio signals emitted by the patient are ultimately translated into a 2-D/3-D image. Gadolinium DTPA, a contrast agent, is commonly injected into the patient to visualize tissue or disease processes.
Two trials funded by NCI to test the promise of MR are being conducted by the International Breast MRI Consortium headed by Mitchell Schnall, M.D., at the University of Pennsylvania (http://www.cancer.gov/clinical_trials). The first is to test the ability of MR to detect cancer and the extent of disease in the breast. Results are expected in the spring of 2002. A second trial will test MR as a screening tool in a pilot study of women at high risk for breast cancer. In addition, the American College of Radiology Imaging Network (ACRIN), a cooperative group funded and supported by NCI that manages clinical trials of imaging technologies as they relate to cancer, is conducting a broad spectrum of multi-institutional clinical trials on new and refined imaging tools. They are planning to test whether MR can improve the ability of conventional methods to stage the extent of disease and to measure treatment response. (http://www.acrin.org)
- Ultrasound
Ultrasound is a technique in which very high frequency sound is directed into the body and reflected back by the tissues. The echoes are picked up and converted into an electrical signal and finally a 2-D or 3-D image. Images are seen in real-time on a television monitor. Photographs of the image can be made for a permanent record.
Kirk Beach, M.D., Ph.D., and his colleagues at the University of Washington in Seattle have constructed a unique ultrasound system that will detect the natural pulsations of tissues. Since tumors larger than 1 mm grow new capillaries to provide an increased blood supply to the tumor, tumors are pulsating more (and therefore have larger volume pulsations) than normal tissue. To detect tumors, the ultrasound machine will look for the areas of larger volume pulsation in the breast representing the greatest density of blood vessels. The investigators hope to detect smaller tumors than can now be detected with mammography. (http://Otir.cancer.gov/tech/uip_awards.html click on ‘awards’)
- Nuclear Medicine
PET (positron emission tomography) and SPECT (single photon emission computed tomography) are nuclear imaging techniques. The images are created by detecting gamma rays produced by man-made radioactive atoms injected into the patient. Gamma detectors move around the patient collecting data that are processed by a computer into a 2-D or 3-D image. The radioactive atoms used in these imaging techniques concentrate selectively in different parts of the body and can be used by themselves or by chemically attaching them to natural substances.
Short-lived positron-emitting atoms are injected into the patient in PET imaging. When positrons travel short distances in tissues and collide with near-by electrons, two gamma- rays are created which travel in opposite directions. The most common positron-emitter, fluorine-18 (half-life 110 minutes), is used to label deoxyglucose, an analogue of glucose. Because tumors metabolize glucose at a faster rate than normal tissue, tumors will take up the radioactive glucose analogue (2-fluoro 2-deoxy-D-glucose, or FDG).
Richard L. Wahl, M.D., the department of radiology at Johns Hopkins University in Baltimore, is evaluating the effectiveness of PET in detecting tumors in dense breasts (breasts that are difficult to image with mammography), and in predicting the outcome of chemotherapy as well as those who will benefit the most from bone marrow transplantation (http://researchportfolio.cancer.gov/ search under breast cancer for ‘imaging’)
- Optical Imaging
Optical imaging refers not only to the use of visible light but also to radiation just beyond the visible — ultraviolet and near-infrared. Several researchers are evaluating the potential of using visible or near infrared light to scan the breast for abnormalities. Britton Chance, Ph.D., D. Sc, at the University of Pennsylvania and Keith Paulsen, Ph.D., and Brian Pogue, Ph.D., at Dartmouth College in New Hampshire are exploring optical detecting methods. (http://researchportfolio.cancer.gov/)
The use of visible probes to detect tumors or molecular markers of precancerous lesions is another area of active research. Ralph Weissleder, M.D., Ph.D., and his colleagues at the Center for Molecular Imaging Research at Massachusetts General Hospital in Boston, one of NCI’s three In Vivo Cellular and Molecular Imaging Centerscreated to encourage the development of clinically useful molecular imaging tools, are developing visible probes that target molecules in tumors. The binding of small molecular probes which are tagged with flurochromes to specific receptors or enzymes in the tumor causes the tumors to give off detectable radiation: http://www3.cancer.gov/bip/icmics.htm.
View photos and slides of different modes of imaging:
Infra-Red imaging: digitphoto.jpg
Optical imaging: optical_imaging.gif
Additional web sites:
Fact Sheet on Improving Methods for Breast Cancer detection and Diagnosis:
http://www.cancer.gov/cancertopics/factsheet/Detection/breast-cancer
NCI’s Division of Extramural Activities: Imaging Sciences Working Group:
http://deainfo.nci.nih.gov/advisory/pog/directors/ director.htm
NCI’s Budget Priorities for Fiscal Year 2002
http://www.cancer.gov/initiatives/grp-image.html
Recent Documents on NCI’s imaging program
http://www3.cancer.gov/bip/news.htm